A galvanic cell, sometimes referred to as a voltaic cell, is a type of electrochemical system that transforms chemical energy into electrical energy via natural redox reactions. These essential power sources are fundamental in modern battery technology, supplying energy for various electronic devices, from handheld torches to advanced smartphones.
In this guide, we explore the structure, working principles, types, and applications of galvanic cell, ensuring a deep understanding of their functionality.
Understanding the Principle of Galvanic Cell
Galvanic cells function by transforming stored chemical energy into electrical energy. This process is driven by a redox reaction, where one material experiences oxidation (electron loss) while another undergoes reduction (electron gain). The disparity in electric potential between these reactions propels the movement of electrons, producing an electrical current.
Components of Galvanic Cell
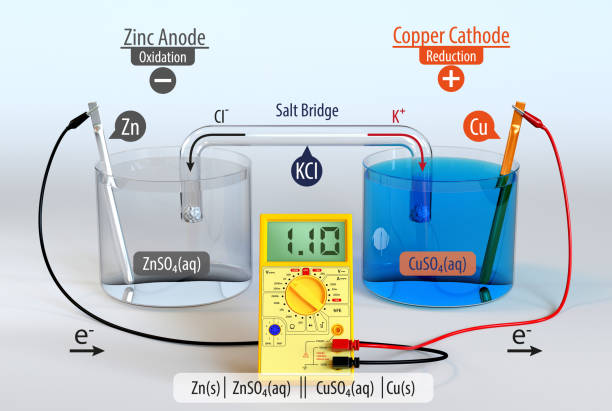
A galvanic cell consists of several essential components:
1. Electrodes
- Anode (-): This is the electrode where oxidation takes place, leading to the release of electrons into the external circuit.
- Cathode (+): The electrode where reduction takes place, gaining electrons.
2. Electrolyte
A solution containing ions that facilitate the redox reaction and redox titration. Each half-cell has its electrolyte, which conducts ions to complete the circuit.
3. Salt Bridge
A U-shaped tube filled with a salt solution (such as KCl or NaNO₃) helps maintain electrical neutrality by permitting the movement of ions between the two half-cells.
4. External Circuit
A conducting wire connects the two electrodes, allowing the flow of electrons from the anode to the cathode.
Example: Daniell Cell
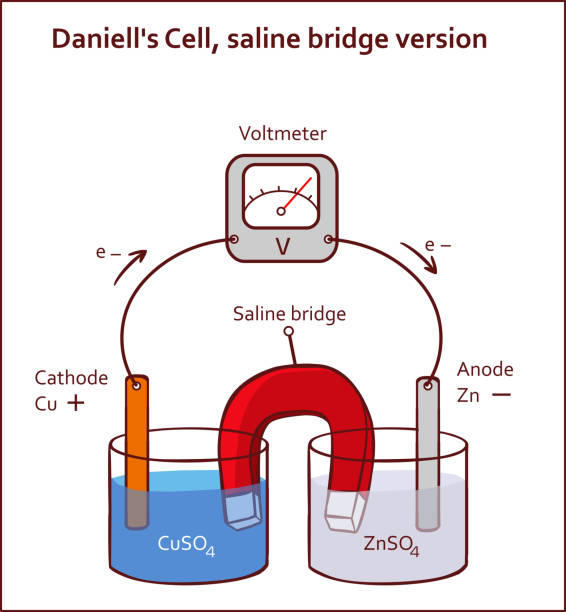
A typical example of a Galvanic cell is the Daniell Cell, which consists of:
- A zinc electrode immersed in zinc sulfate (ZnSO₄) solution (anode).
- A copper electrode immersed in copper sulfate (CuSO₄) solution (cathode).
- A salt bridge connecting the two solutions.
Working Mechanism of Galvanic Cell
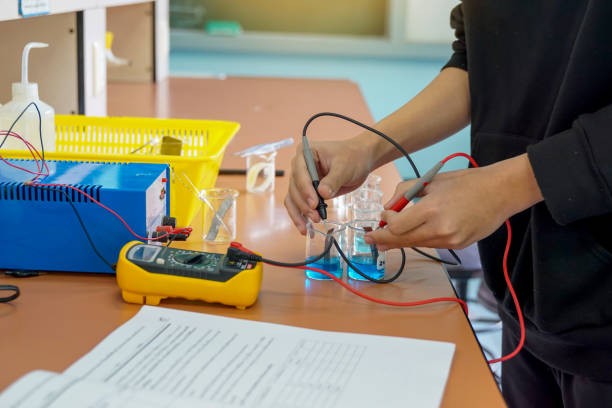
Redox reactions govern the operation of galvanic cells. Let’s consider a zinc-copper (Zn-Cu) galvanic cells as an example:
- Oxidation at the Anode:
- Zinc (Zn) loses electrons: Zn(s) → Zn²⁺(aq) + 2e⁻.
- Electrons travel through the external circuit to the cathode.
- Reduction at the Cathode:
- Copper (Cu²⁺) gains electrons: Cu²⁺(aq) + 2e⁻ → Cu(s).
- Solid copper is deposited on the cathode.
- Ion Movement Through the Salt Bridge:
- Negative ions (e.g., Cl⁻) move toward the anode to neutralize Zn²⁺ ions.
- Positive ions (e.g., K⁺) migrate toward the cathode to balance the loss of Cu²⁺.
The continuous electron flow from the anode to the cathode generates an electric current.
Electrode Potential and Voltage in Galvanic Cell
The electromotive force (EMF) or cell voltage (E° cell) represents the electrical potential difference produced by a galvanic cell. This value is determined based on standard electrode potentials:
E°cell = E°cathode – E°anode.
For a Zn-Cu cell:
- E° (Cu²⁺/Cu) = +0.34V
- E° (Zn²⁺/Zn) = -0.76V
- E° cell = (0.34V) – (-0.76V) = 1.10V
A positive E° cell indicates a spontaneous reaction, meaning the cell generates electricity naturally.
Types of Galvanic Cell
1. Daniel Cell
- Uses Zn and Cu electrodes with ZnSO₄ and CuSO₄ electrolytes.
- Generates 1.10V.
2. Dry Cell (Leclanché Cell)
- Common in flashlights and remote controls.
- Uses a zinc anode, manganese dioxide cathode, and ammonium chloride electrolyte.
3. Lead-Acid Battery
- Found in automobiles.
- Uses lead (Pb) and lead dioxide (PbO₂) electrodes with sulfuric acid (H₂SO₄) as the electrolyte.
4. Lithium-Ion Battery
- Used in smartphones and laptops.
- Contains lithium-based electrodes and an organic electrolyte.
Applications of Galvanic Cell
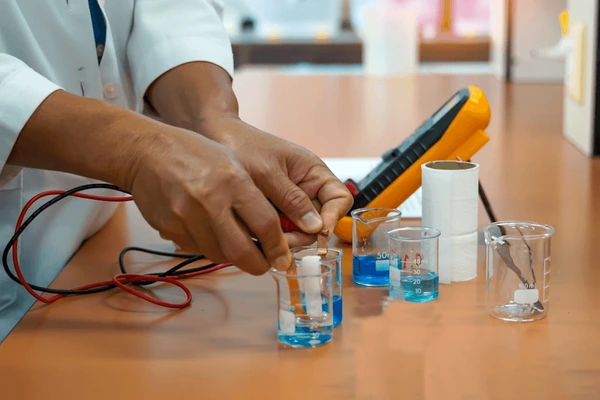
- Batteries: Power sources for portable electronics and vehicles.
- Corrosion Prevention: Used in cathodic protection for metal structures.
- Metal Refining: Electrochemical deposition in electroplating industries.
- Biological Systems: Nerve signal transmissions in living organisms.
- Fuel Cells: Advanced fuel cells convert chemical energy from hydrogen into electrical energy.
- Electrochemical Sensors: Devices like pH meters use Galvanic cell mechanisms to measure ion concentrations.
Factors Affecting Galvanic Cells Performance
- Concentration of Electrolyte: Higher ion concentration increases voltage.
- Temperature: Affects reaction kinetics and EMF.
- Electrode Surface Area: A larger surface area enhances reaction rates.
- Nature of Electrodes: Different metals have different standard reduction potentials.
Conclusion
Galvanic cells are essential in everyday life, from simple batteries to complex energy storage systems. Understanding their principles helps in optimizing their use for sustainable energy solutions.
Galvanic cells are fundamental to modern electrochemistry, providing sustainable solutions for energy storage and industrial applications. By understanding their principles, working mechanisms, and applications, scientists and engineers can innovate and improve various technologies for a more efficient future.
Stay tuned for more insights into electrochemical processes and their significance in everyday life!
How does Galvanic Cells Work?
Working Mechanism of Galvanic Cells
Redox reactions govern the operation of galvanic cells. Let’s consider a zinc-copper (Zn-Cu) galvanic cells as an example:
Oxidation at the Anode: Zinc (Zn) loses electrons: Zn(s) → Zn²⁺(aq) + 2e⁻.
Electrons travel through the external circuit to the cathode.
Reduction at the Cathode: Copper (Cu²⁺) gains electrons: Cu²⁺(aq) + 2e⁻ → Cu(s).
Solid copper is deposited on the cathode.
Ion Movement Through the Salt Bridge: Negative ions (e.g., Cl⁻) move toward the anode to neutralize Zn²⁺ ions.
Positive ions (e.g., K⁺) migrate toward the cathode to balance the loss of Cu²⁺.
The continuous electron flow from the anode to the cathode generates an electric current.
A galvanics cell, sometimes referred to as a voltaic cell, is a type of electrochemical system that transforms chemical energy into electrical energy via natural redox reactions. These essential power sources are fundamental in modern battery technology, supplying energy for various electronic devices, from handheld torches to advanced smartphones.
In this guide, we explore the structure, working principles, types, and applications of galvanic cells, ensuring a deep understanding of their functionality.
Understanding the Principle of Galvanic Cells
Galvanics cells function by transforming stored chemical energy into electrical energy. This process is driven by a redox reaction, where one material experiences oxidation (electron loss) while another undergoes reduction (electron gain). The disparity in electric potential between these reactions propels the movement of electrons, producing an electrical current.
Components of Galvanics Cells
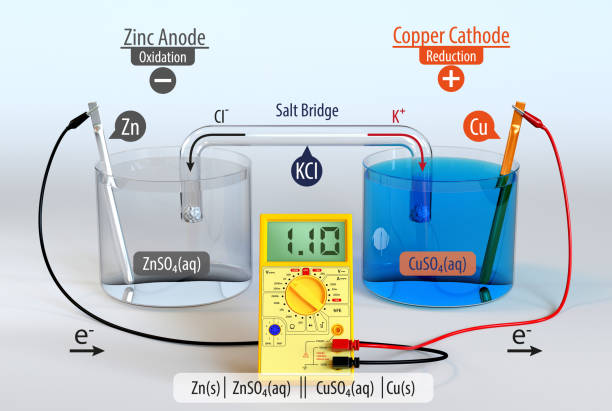
A galvanics cell consists of several essential components:
1. Electrodes
Anode (-): This is the electrode where oxidation takes place, leading to the release of electrons into the external circuit.
Cathode (+): The electrode where reduction takes place, gaining electrons.
2. Electrolyte
A solution containing ions that facilitate the redox reaction and redox titration. Each half-cell has its electrolyte, which conducts ions to complete the circuit.
3. Salt Bridge
A U-shaped tube filled with a salt solution (such as KCl or NaNO₃) helps maintain electrical neutrality by permitting the movement of ions between the two half-cells.
4. External Circuit
A conducting wire connects the two electrodes, allowing the flow of electrons from the anode to the cathode.
Example: Daniell Cell
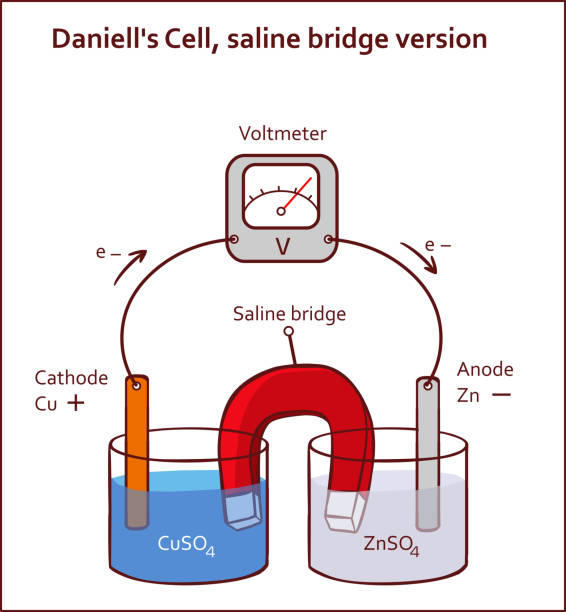
A typical example of a Galvanics cell is the Daniell Cell, which consists of:
A zinc electrode immersed in zinc sulfate (ZnSO₄) solution (anode).
A copper electrode immersed in copper sulfate (CuSO₄) solution (cathode).
A salt bridge connecting the two solutions.
Working Mechanism of Galvanics Cells
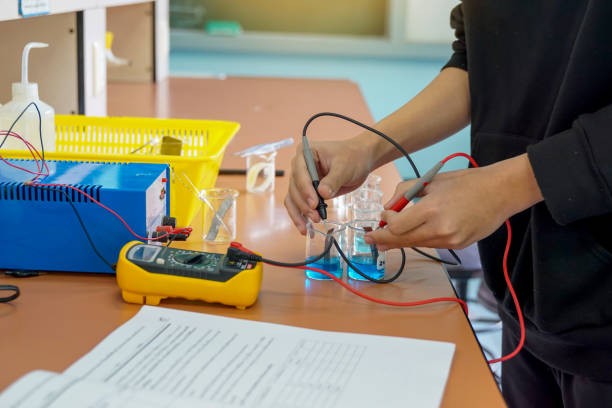
Redox reactions govern the operation of galvanics cells. Let’s consider a zinc-copper (Zn-Cu) galvanics cells as an example:
Oxidation at the Anode: Zinc (Zn) loses electrons: Zn(s) → Zn²⁺(aq) + 2e⁻.
Electrons travel through the external circuit to the cathode.
Reduction at the Cathode: Copper (Cu²⁺) gains electrons: Cu²⁺(aq) + 2e⁻ → Cu(s).
Solid copper is deposited on the cathode.
Ion Movement Through the Salt Bridge: Negative ions (e.g., Cl⁻) move toward the anode to neutralize Zn²⁺ ions.
Positive ions (e.g., K⁺) migrate toward the cathode to balance the loss of Cu²⁺.
The continuous electron flow from the anode to the cathode generates an electric current.
Electrode Potential and Voltage in Galvanic Cells
The electromotive force (EMF) or cell voltage (E° cell) represents the electrical potential difference produced by a galvanic cell. This value is determined based on standard electrode potentials:
E°cell = E°cathode – E°anode.
For a Zn-Cu cell:
E° (Cu²⁺/Cu) = +0.34V
E° (Zn²⁺/Zn) = -0.76V
E° cell = (0.34V) – (-0.76V) = 1.10V
A positive E° cell indicates a spontaneous reaction, meaning the cell generates electricity naturally.
Types of Galvanic Cells
1. Daniel Cell
Uses Zn and Cu electrodes with ZnSO₄ and CuSO₄ electrolytes.
Generates 1.10V.
2. Dry Cell (Leclanché Cell)
Common in flashlights and remote controls.
Uses a zinc anode, manganese dioxide cathode, and ammonium chloride electrolyte.
3. Lead-Acid Battery
Found in automobiles.
Uses lead (Pb) and lead dioxide (PbO₂) electrodes with sulfuric acid (H₂SO₄) as the electrolyte.
4. Lithium-Ion Battery
Used in smartphones and laptops.
Contains lithium-based electrodes and an organic electrolyte.
Applications of Galvanic Cells
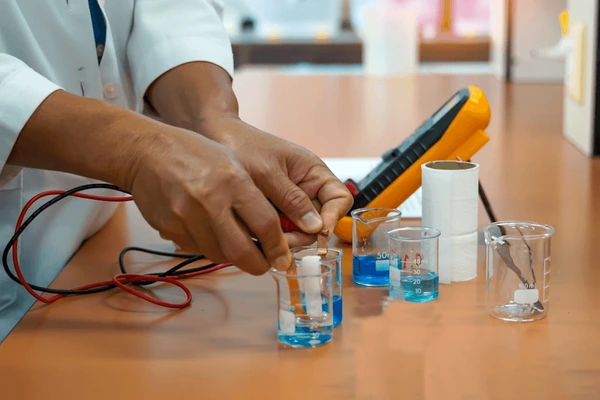
Batteries: Power sources for portable electronics and vehicles.
Corrosion Prevention: Used in cathodic protection for metal structures.
Metal Refining: Electrochemical deposition in electroplating industries.
Biological Systems: Nerve signal transmissions in living organisms.
Fuel Cells: Advanced fuel cells convert chemical energy from hydrogen into electrical energy.
Electrochemical Sensors: Devices like pH meters use Galvanic cell mechanisms to measure ion concentrations.
Factors Affecting Galvanic Cells Performance
Concentration of Electrolyte: Higher ion concentration increases voltage.
Temperature: Affects reaction kinetics and EMF.
Electrode Surface Area: A larger surface area enhances reaction rates.
Nature of Electrodes: Different metals have different standard reduction potentials.
Conclusion
Galvanic cells are essential in everyday life, from simple batteries to complex energy storage systems. Understanding their principles helps in optimizing their use for sustainable energy solutions.
Galvanic cells are fundamental to modern electrochemistry, providing sustainable solutions for energy storage and industrial applications. By understanding their principles, working mechanisms, and applications, scientists and engineers can innovate and improve various technologies for a more efficient future.
What is a galvanic cell Class 12?
In this guide, we explore the structure, working principles, types, and applications of galvanic cells, ensuring a deep understanding of their functionality.
Understanding the Principle of Galvanic Cells
Galvanic cells function by transforming stored chemical energy into electrical energy. This process is driven by a redox reaction, where one material experiences oxidation (electron loss) while another undergoes reduction (electron gain). The disparity in electric potential between these reactions propels the movement of electrons, producing an electrical current.
Components of Galvanic Cells
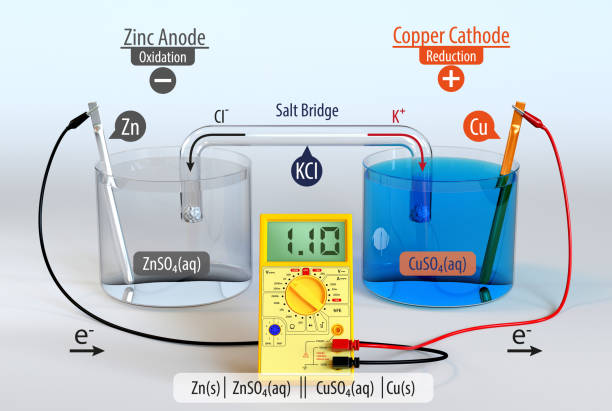
A galvanic cell consists of several essential components:
1. Electrodes
Anode (-): This is the electrode where oxidation takes place, leading to the release of electrons into the external circuit.
Cathode (+): The electrode where reduction takes place, gaining electrons.
2. Electrolyte
A solution containing ions that facilitate the redox reaction and redox titration. Each half-cell has its electrolyte, which conducts ions to complete the circuit.
3. Salt Bridge
A U-shaped tube filled with a salt solution (such as KCl or NaNO₃) helps maintain electrical neutrality by permitting the movement of ions between the two half-cells.
4. External Circuit
A conducting wire connects the two electrodes, allowing the flow of electrons from the anode to the cathode.
Example: Daniell Cell
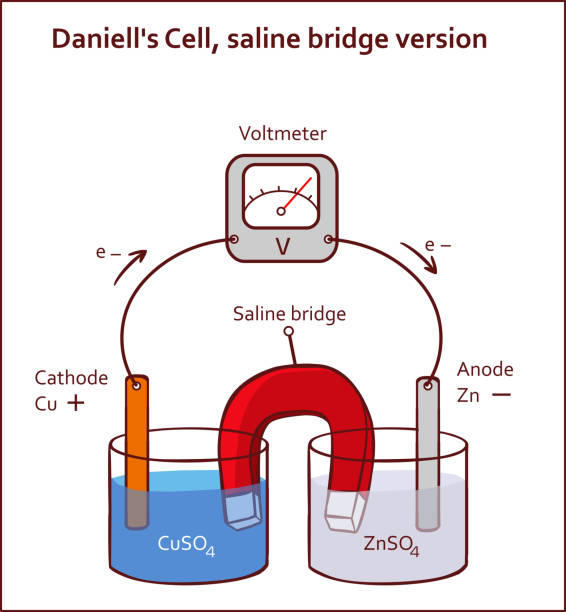
A typical example of a Galvanic cell is the Daniell Cell, which consists of:
A zinc electrode immersed in zinc sulfate (ZnSO₄) solution (anode).
A copper electrode immersed in copper sulfate (CuSO₄) solution (cathode).
A salt bridge connecting the two solutions.
Working Mechanism of Galvanic Cells
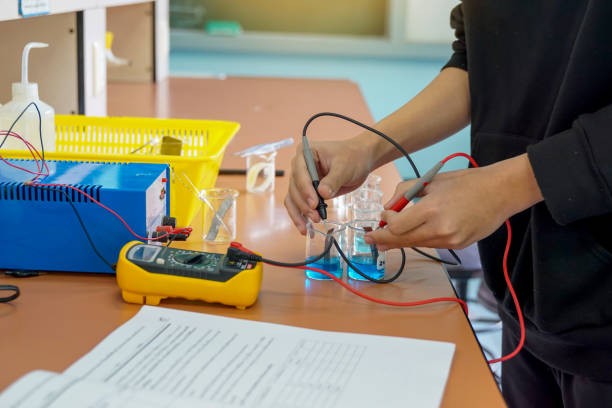
Redox reactions govern the operation of galvanic cells. Let’s consider a zinc-copper (Zn-Cu) galvanic cells as an example:
Oxidation at the Anode: Zinc (Zn) loses electrons: Zn(s) → Zn²⁺(aq) + 2e⁻.
Electrons travel through the external circuit to the cathode.
Reduction at the Cathode: Copper (Cu²⁺) gains electrons: Cu²⁺(aq) + 2e⁻ → Cu(s).
Solid copper is deposited on the cathode.
Ion Movement Through the Salt Bridge: Negative ions (e.g., Cl⁻) move toward the anode to neutralize Zn²⁺ ions.
Positive ions (e.g., K⁺) migrate toward the cathode to balance the loss of Cu²⁺.
The continuous electron flow from the anode to the cathode generates an electric current.
Electrode Potential and Voltage in Galvanic Cells
The electromotive force (EMF) or cell voltage (E° cell) represents the electrical potential difference produced by a galvanic cell. This value is determined based on standard electrode potentials:
E°cell = E°cathode – E°anode.
For a Zn-Cu cell:
E° (Cu²⁺/Cu) = +0.34V
E° (Zn²⁺/Zn) = -0.76V
E° cell = (0.34V) – (-0.76V) = 1.10V
A positive E° cell indicates a spontaneous reaction, meaning the cell generates electricity naturally.
Types of Galvanic Cells
1. Daniel Cell
Uses Zn and Cu electrodes with ZnSO₄ and CuSO₄ electrolytes.
Generates 1.10V.
2. Dry Cell (Leclanché Cell)
Common in flashlights and remote controls.
Uses a zinc anode, manganese dioxide cathode, and ammonium chloride electrolyte.
3. Lead-Acid Battery
Found in automobiles.
Uses lead (Pb) and lead dioxide (PbO₂) electrodes with sulfuric acid (H₂SO₄) as the electrolyte.
4. Lithium-Ion Battery
Used in smartphones and laptops.
Contains lithium-based electrodes and an organic electrolyte.
Applications of Galvanic Cells
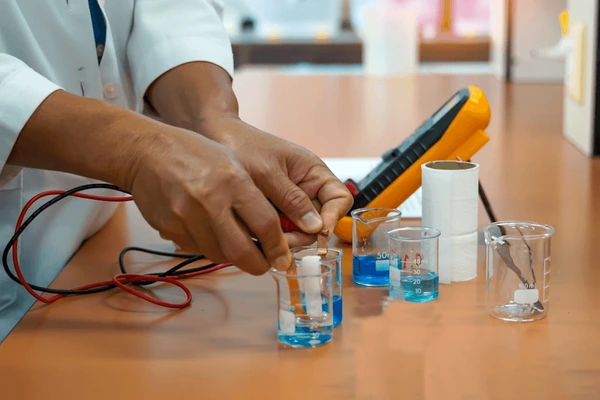
Batteries: Power sources for portable electronics and vehicles.
Corrosion Prevention: Used in cathodic protection for metal structures.
Metal Refining: Electrochemical deposition in electroplating industries.
Biological Systems: Nerve signal transmissions in living organisms.
Fuel Cells: Advanced fuel cells convert chemical energy from hydrogen into electrical energy.
Electrochemical Sensors: Devices like pH meters use Galvanic cell mechanisms to measure ion concentrations.
Factors Affecting Galvanic Cells Performance
Concentration of Electrolyte: Higher ion concentration increases voltage.
Temperature: Affects reaction kinetics and EMF.
Electrode Surface Area: A larger surface area enhances reaction rates.
Nature of Electrodes: Different metals have different standard reduction potentials.
Conclusion
Galvanic cells are essential in everyday life, from simple batteries to complex energy storage systems. Understanding their principles helps in optimizing their use for sustainable energy solutions.
Galvanic cells are fundamental to modern electrochemistry, providing sustainable solutions for energy storage and industrial applications. By understanding their principles, working mechanisms, and applications, scientists and engineers can innovate and improve various technologies for a more efficient future.
What is the difference between Electrolytic Cells and galvanic cells?
Feature | Galvanic Cell | Electrolytic Cell |
---|---|---|
Energy Source | Chemical reaction | External power source |
Reaction Type | Spontaneous | Non-spontaneous |
Electrode Charges | Anode (-), Cathode (+) | Anode (+), Cathode (-) |
Purpose | Generate electricity | Drive chemical reactions |
Example | Batteries | Electroplating |
What is called a voltaic cell?
In this guide, we explore the structure, working principles, types, and applications of galvanic cells, ensuring a deep understanding of their functionality.
Understanding the Principle of Galvanic Cells
Galvanic cells function by transforming stored chemical energy into electrical energy. This process is driven by a redox reaction, where one material experiences oxidation (electron loss) while another undergoes reduction (electron gain). The disparity in electric potential between these reactions propels the movement of electrons, producing an electrical current.
Components of Galvanic Cells
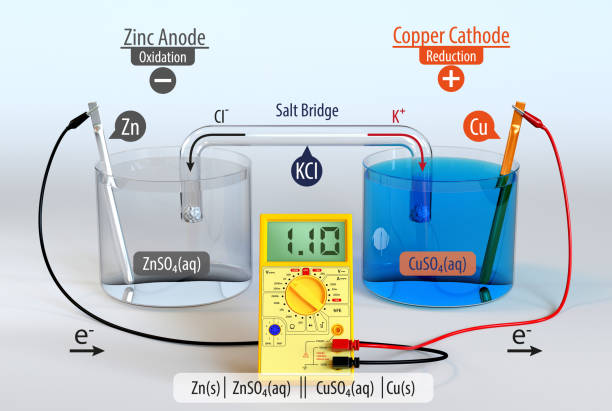
A galvanic cell consists of several essential components:
1. Electrodes
Anode (-): This is the electrode where oxidation takes place, leading to the release of electrons into the external circuit.
Cathode (+): The electrode where reduction takes place, gaining electrons.
2. Electrolyte
A solution containing ions that facilitate the redox reaction and redox titration. Each half-cell has its electrolyte, which conducts ions to complete the circuit.
3. Salt Bridge
A U-shaped tube filled with a salt solution (such as KCl or NaNO₃) helps maintain electrical neutrality by permitting the movement of ions between the two half-cells.
4. External Circuit
A conducting wire connects the two electrodes, allowing the flow of electrons from the anode to the cathode.
Example: Daniell Cell
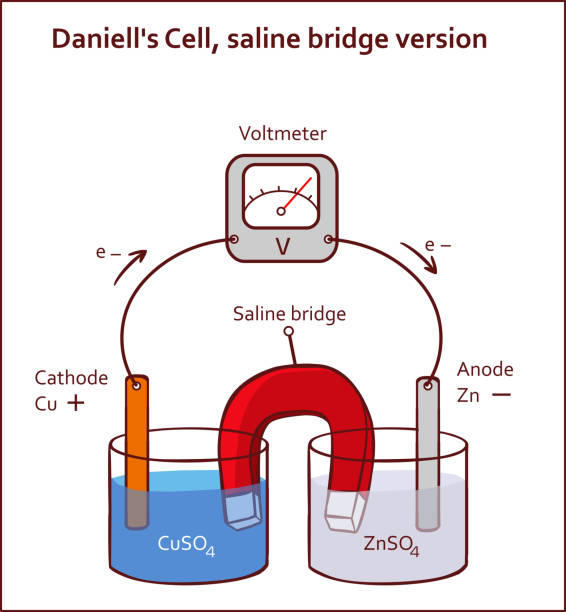
A typical example of a Galvanic cell is the Daniell Cell, which consists of:
A zinc electrode immersed in zinc sulfate (ZnSO₄) solution (anode).
A copper electrode immersed in copper sulfate (CuSO₄) solution (cathode).
A salt bridge connecting the two solutions.
Working Mechanism of Galvanic Cells
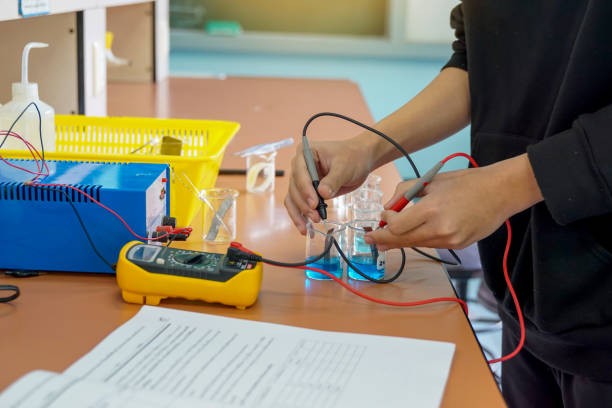
Redox reactions govern the operation of galvanic cells. Let’s consider a zinc-copper (Zn-Cu) galvanic cells as an example:
Oxidation at the Anode: Zinc (Zn) loses electrons: Zn(s) → Zn²⁺(aq) + 2e⁻.
Electrons travel through the external circuit to the cathode.
Reduction at the Cathode: Copper (Cu²⁺) gains electrons: Cu²⁺(aq) + 2e⁻ → Cu(s).
Solid copper is deposited on the cathode.
Ion Movement Through the Salt Bridge: Negative ions (e.g., Cl⁻) move toward the anode to neutralize Zn²⁺ ions.
Positive ions (e.g., K⁺) migrate toward the cathode to balance the loss of Cu²⁺.
The continuous electron flow from the anode to the cathode generates an electric current.
Electrode Potential and Voltage in Galvanic Cells
The electromotive force (EMF) or cell voltage (E° cell) represents the electrical potential difference produced by a galvanic cell. This value is determined based on standard electrode potentials:
E°cell = E°cathode – E°anode.
For a Zn-Cu cell:
E° (Cu²⁺/Cu) = +0.34V
E° (Zn²⁺/Zn) = -0.76V
E° cell = (0.34V) – (-0.76V) = 1.10V
A positive E° cell indicates a spontaneous reaction, meaning the cell generates electricity naturally.
Types of Galvanic Cells
1. Daniel Cell
Uses Zn and Cu electrodes with ZnSO₄ and CuSO₄ electrolytes.
Generates 1.10V.
2. Dry Cell (Leclanché Cell)
Common in flashlights and remote controls.
Uses a zinc anode, manganese dioxide cathode, and ammonium chloride electrolyte.
3. Lead-Acid Battery
Found in automobiles.
Uses lead (Pb) and lead dioxide (PbO₂) electrodes with sulfuric acid (H₂SO₄) as the electrolyte.
4. Lithium-Ion Battery
Used in smartphones and laptops.
Contains lithium-based electrodes and an organic electrolyte.
Applications of Galvanic Cells
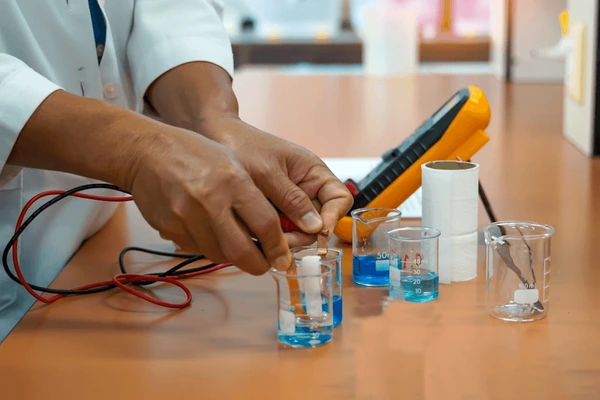
Batteries: Power sources for portable electronics and vehicles.
Corrosion Prevention: Used in cathodic protection for metal structures.
Metal Refining: Electrochemical deposition in electroplating industries.
Biological Systems: Nerve signal transmissions in living organisms.
Fuel Cells: Advanced fuel cells convert chemical energy from hydrogen into electrical energy.
Electrochemical Sensors: Devices like pH meters use Galvanic cell mechanisms to measure ion concentrations.
Factors Affecting Galvanic Cells Performance
Concentration of Electrolyte: Higher ion concentration increases voltage.
Temperature: Affects reaction kinetics and EMF.
Electrode Surface Area: A larger surface area enhances reaction rates.
Nature of Electrodes: Different metals have different standard reduction potentials.
Conclusion
Galvanic cells are essential in everyday life, from simple batteries to complex energy storage systems. Understanding their principles helps in optimizing their use for sustainable energy solutions.
Galvanic cells are fundamental to modern electrochemistry, providing sustainable solutions for energy storage and industrial applications. By understanding their principles, working mechanisms, and applications, scientists and engineers can innovate and improve various technologies for a more efficient future.
What are the applications of galvanic cells?
Galvanic cells have various real-world applications, including:
Batteries: Primary and secondary batteries, such as alkaline and lithium-ion batteries, function based on Galvanic cell principles.
Corrosion Protection: Sacrificial anodes in ships and pipelines use Galvanic cells to prevent rusting.
Fuel Cells: Advanced fuel cells convert chemical energy from hydrogen into electrical energy.
Electrochemical Sensors: Devices like pH meters use Galvanic cell mechanisms to measure ion concentrations.
What are the types of Galvanic Cells?
1. Daniel Cell
Uses Zn and Cu electrodes with ZnSO₄ and CuSO₄ electrolytes.
Generates 1.10V.
2. Dry Cell (Leclanché Cell)
Common in flashlights and remote controls.
Uses a zinc anode, manganese dioxide cathode, and ammonium chloride electrolyte.
3. Lead-Acid Battery
Found in automobiles.
Uses lead (Pb) and lead dioxide (PbO₂) electrodes with sulfuric acid (H₂SO₄) as the electrolyte.
4. Lithium-Ion Battery
Used in smartphones and laptops.
Contains lithium-based electrodes and an organic electrolyte.
What are the factors affecting Galvanic Cells’ performance?
Concentration of Electrolyte: Higher ion concentration increases voltage.
Temperature: Affects reaction kinetics and EMF.
Electrode Surface Area: A larger surface area enhances reaction rates.
Nature of Electrodes: Different metals have different standard reduction potentials.
What are the components of Galvanic Cells?
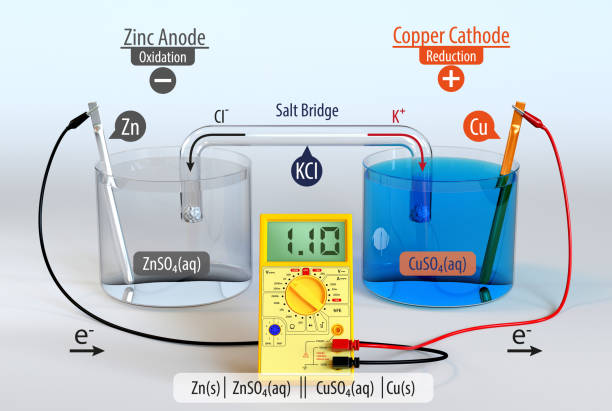
A galvanic cell consists of several essential components:
1. Electrodes
Anode (-): This is the electrode where oxidation takes place, leading to the release of electrons into the external circuit.
Cathode (+): The electrode where reduction takes place, gaining electrons.
2. Electrolyte
A solution containing ions that facilitate the redox reaction and redox titration. Each half-cell has its electrolyte, which conducts ions to complete the circuit.
3. Salt Bridge
A U-shaped tube filled with a salt solution (such as KCl or NaNO₃) helps maintain electrical neutrality by permitting the movement of ions between the two half-cells.
4. External Circuit
A conducting wire connects the two electrodes, allowing the flow of electrons from the anode to the cathode.
Example: Daniell Cell
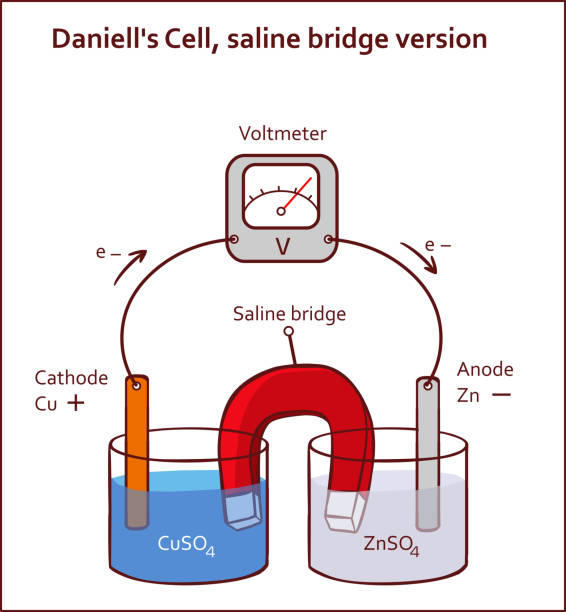
A typical example of a Galvanic cell is the Daniell Cell, which consists of:
A zinc electrode immersed in zinc sulfate (ZnSO₄) solution (anode).
A copper electrode immersed in copper sulfate (CuSO₄) solution (cathode).
A salt bridge connecting the two solutions.